Auteur: Prof. dr. Ad van Wijk
Dossier: De opmars van waterstof
Gasthoofdredacteur: Prof. dr. Ad van Wijk
Summary
Hydrogen and electricity are energy carriers that can be made from fossil and renewable energy. Green hydrogen can be produced from water and green electricity through water electrolysis.
Worldwide, the potential for solar and wind energy is huge. Only 8% of the Sahara Desert area, if covered with solar panels, could produce all of the global energy demand. Electricity from solar and wind is cheap in places with high solar irradiation or wind speeds, at locations often far away from demand centres. To supply cheap solar and wind electricity at the right place and at all times, hydrogen is necessary because it is much cheaper to transport and store than electricity. A smart combination of green electricity and green hydrogen leads to a more reliable system with overall lower system cost compared to a system without hydrogen.Therefore, a sustainable energy system will have two very important symbiotic energy carriers; electricity and hydrogen. Electricity and hydrogen can be converted into each other using proven electro-chemical conversion technologies; electrolysers and fuel cells. After the steam era and combustion era, it is now time for the Electro-Hydrogen era!
What is hydrogen: grey, blue and green hydrogen
Like electricity, hydrogen is an energy carrier that can be made from renewable energy sources, but also from fossil energy sources. But hydrogen, like electricity, is not an energy source!
Hydrogen from fossil hydrocarbons
Hydrocarbons or fossil fuels are compounds consisting of carbon and hydrogen. For example, methane (CH4) the main component of natural gas, is a compound of one carbon atom with 4 hydrogen atoms. The hydrogen atoms can be split from the carbon atoms using various techniques, including the widely used Steam Methane Reforming (SMR). Steam (H2O) reacts with methane (CH4) to form hydrogen (H2) and carbon dioxide (CO2), depicted in the following reactions:
CH4 + H2O (steam) ? 3H2 + CO
CO + H2O (steam) ? H2 + CO2
As can be seen, carbon dioxide CO2 is formed here. If the CO2 is emitted into the air, contributing to the greenhouse effect, the resulting hydrogen is called grey hydrogen.
The CO2, which is generated in pure form, can also be captured and stored in an empty gas field or aquifer. This is called Carbon Capture and Storage or CCS. Up to 90% of the CO2 can be captured and stored in this way. Hydrogen produced through SMR in combination with CCS is called low-carbon hydrogen or blue hydrogen.
Hydrogen from bio hydrocarbons
Biogas or synthesis gas can be made from organic residues such as manure, waste wood or organic waste. Such syngas consists of various components: methane, carbon dioxide, carbon monoxide, but also to a greater or lesser extent hydrogen. Pure Hydrogen with pure carbon dioxide can be made from biogas/syngas. If the share of methane is high, this can be converted into hydrogen and carbon dioxide using SMR as described above. Since the feedstock is green, this process yields green hydrogen and green carbon dioxide.
Both green hydrogen and green carbon (dioxide) are valuable because they can be used as feedstock to produce green chemicals or green fuels such as plastics or green synthetic kerosene. Because the process replaces fossil carbon with carbon captured from the atmosphere when the biomass grows, CO2 emissions are avoided. Better still, when this green carbon dioxide is captured and stored, the net greenhouse gas emissions are negative.
Hydrogen from water
Hydrogen can also be made from water (H2O) in a process called electrolysis, which is a chemical reaction in which a substance is decomposed into components under the influence of an electric current. In water electrolysis, the water molecule (H2O) is split into hydrogen (H2) and oxygen (O2), depicted in the following reaction:
Electrolyser: 2H2O + 2e– ? 2H2 + O2
If the electricity is produced in a coal, oil or gas power plant, the hydrogen is not carbon free and is called grey hydrogen. But if the electricity is generated using green electricity from solar panels, wind turbines or hydroelectric power, the hydrogen is called green hydrogen.
It is the energy source that determines whether the hydrogen is green or not.
Worldwide energy consumption.
About 90% of the world’s energy consumption in 2016, amounting to 155,000 TWh (TWh=billion kWh), is fossil energy: oil, gas and coal. For comparison, the Netherlands uses about 1,000 TWh per year. This fossil energy is transported around the world by ship or pipeline, then converted into a useful energy carrier, electricity, gasoline/diesel or a gas. The conversion to a useful energy carrier is often associated with energy losses. For example, the efficiency of a modern gas-fired power plant is about 60%, which implies that 40% of the energy is lost as heat. These useful energy carriers are then distributed and used in houses, cars, factories, etc.
Energy is used in many parts of our modern life: For heating and cooling houses and buildings. For electricity production to power equipment, appliances and lighting. For transport by vehicles, ships or planes. And for industry, where processes require high temperature heat and steam. But fossil energy is also a feedstock, from which chemical products such as plastics or fertilizers can be made.
Hydrogen is not only an energy carrier but also a feedstock. Hydrogen is nowadays mainly made from natural gas or coal and primarily used as a feedstock to produce ammonia (the main component of fertilizers) or methanol, among other things. And hydrogen is used in refineries to desulfurize oil and in the production of gasoline and diesel.
Can solar and wind energy replace all fossil energy?
The question is whether all the fossil energy used worldwide can be replaced by green energy, and is it possible to generate it using solar panels and wind turbines. The answer is simply: no problem. The potential of solar and wind energy is very large.
If worldwide energy demand, amounting to 155,000 TWh, had to be produced with solar panels only, it would require a surface area covering about 10% of Australia or 8% of the Sahara Desert. The Sahara Desert is about 9.2 million square kilometres in size, which is more than twice the area covered by the European Union.
The global wind energy potential is also very high. In a scenario where the entire worldwide energy demand would be produced with wind turbines, would only requires an area of 1.5% of the Pacific Ocean. It should be noted that that surface, however, is used to a limited extent, with one large floating wind turbine every kilometer.
So there is more than enough space to produce all the necessary energy for the whole world with solar and wind. This is even the case with an increasing global population and rising prosperity level.
Why is hydrogen needed?
Clean electricity is produced by solar panels and wind turbines. Electricity can be used to power all kinds of devices and for lighting, but also for heating, cooling and for mobility. So why would we convert electricity into hydrogen, with energy losses and extra costs?
Solar panels can produce electricity very cheaply in locations where solar irradiation is highest, for example in desert areas. The lowest prices for electricity from solar panels that have been offered in large tenders recently, April 2020, are around 1.25 and 1.5 Euro cents per kWh.
Wind turbines can also produce electricity very cheaply in windy places, mainly at sea and in certain areas such as Patagonia or Kazakhstan. But it can also be very windy in desert areas, also in the Sahara Desert, around the Red Sea or in areas in Algeria and Morocco. Electricity production cost with wind turbines on land are, mid 2020, around 2 Euro cents per kWh at good sites.
Today, in 2020, solar and wind electricity is even cheaper than electricity generated using fossil fuels, but mainly in areas far away from the demand. That leaves the question how this cheap green energy can be delivered to the energy user at the right place and time. This requires transport and storage of energy. Transport of electricity is possible via electricity cables, but that is roughly 10 times more expensive than transporting the same amount of energy as hydrogen by pipeline. Equally, storage of electricity in batteries or by pumping up water is more than 100 times as expensive than storing hydrogen in underground salt caverns.
For longer distances, beyond the feasible range of cables or pipelines, energy will have to be transported by train, truck or ship. The only feasible pathway is by converting electricity into hydrogen and convert it for transport. Like natural gas, hydrogen can be liquefied, which requires temperatures of -253 degrees Celsius, only 20 degrees above the absolute zero. Hydrogen can also be combined with nitrogen from air to produce ammonia, the main component of fertilizer, which is already liquid at -33 degrees Celsius, so cheaper and easier to transport than liquid hydrogen.
Now comes calculating, what is cheaper? Is it cheaper to generate electricity from solar or wind far away from demand and to bring it to the user via electricity cables and when necessary store this electricity in batteries? Or is a conversion to hydrogen and transport by pipeline and hydrogen storage in salt caverns and (if necessary) conversion back to electricity cheaper, despite the energy losses and extra conversion cost? Or is higher cost solar or wind electricity generation closer to the user, but with lower transport costs, a cheaper solution?
An example for a simplified comparison is given in the diagram below. It is a comparison between a solar cell system on the roof of a house in North-Western Europe and a solar cell system in a desert. Both systems must supply 100 kWh electricity to the house. The assumption is that desert electricity can be produced at a cost of 1 Eurocent per kWh. Because the yield at the roof of a house in North-Western Europe is a factor 2 to 3 lower and the costs of a small system are a factor 2.5 to 3 higher than for a large system in the desert, the solar panels on the house produce electricity at a cost of 5-9 Euro cents per kWh. So, 100 kWh electricity from the solar panels on the roof costs 5-9 Euro.
The solar power from the desert, on the other hand, is converted via electrolysis into hydrogen, liquefied and transported in a ship, put in a pipeline and converted into electricity at home with a fuel cell. Electrolysis in the desert requires not only solar electricity but also water. By pipeline, sea water can be transported to these solar hydrogen production plants, reverse osmosis converts the sea water into demineralized water, the feedstock for hydrogen production. Even with distances over 1.000 km, these demineralized water cost, are only a couple percent of total hydrogen production cost.
Despite all the extra costs and losses, 100 kWh of solar power from the desert costs about the same as that from the solar panels on the roof. The major advantage of the desert electricity, however, is the availability at any time, summer and winter and day and night.
It is also interesting to note that a factor of 2.5 more electricity must be produced in the desert given the conversion losses, but the number of solar panels placed on the roof or in the desert is approximately the same. After all, the same solar panel in the desert produces 2-3 times as much as on the roof in Europe.
This example shows that a sustainable energy system is about total system costs and not about system efficiency. And it clearly explains the merit of hydrogen in a sustainable energy system, namely for cheap transport and storage of low cost solar and wind electricity. With hydrogen, a clean, affordable and reliable sustainable energy system can be realized. And green hydrogen, along with green electricity, can easily replace all fossil fuels.
Electricity and hydrogen symbiosis; The electro-hydrogen era!
A global sustainable energy system can be constructed with mainly solar and wind as energy source, converted into electricity via solar panels and wind turbines. Where possible, useful and cost effective, the electricity produced is directly used. However, lowest cost solar and wind electricity can also be produced far away from the demand, requiring conversion to hydrogen for cheap transport and storage. The lower electricity production cost and cheaper transport and storage cost will compensate for extra energy conversion losses and costs.
Hydrogen as a feedstock to produce chemical products is a direct use of hydrogen. But the application of hydrogen for heating, mobility and power means that hydrogen needs to be converted again into electricity or heat. Today, the conversion is via combustion in a boiler, furnace, gas engine or turbine. However, in future the electrochemical conversion via fuel cells will become more important. The fuel cell reaction is the reverse of the electrolyser reaction: hydrogen and oxygen form water and the process produces electricity:
Fuel cell: 2H2 + O2 ? 2H2O + 2e–
Fuel cells that produce electricity and heat will be used in houses and buildings. The volume and temperature level of the heat can be brought to the desired level by using heat pumps. And the electricity from the fuel cells supplements the electricity from solar panels on the roof.
In future, electricity from batteries can directly power an electric engine for light cars, ships and airplanes, that travel short distances. For heavier vehicles, ships, trains and airplanes with longer ranges, an onboard fuel cell converts hydrogen into electricity, which drives the electric motor. Much more energy can be carried on board by hydrogen (gaseous, liquid or packaged as e.g. ammonia) than by electricity in batteries.
In a transitional period, hydrogen can also be used for heating, mobility and electricity production, by combusting it in a boiler, motor or turbine. But eventually electrochemical conversion using electrolysers and fuel cells will dominate. Electricity and hydrogen will be the carbon-free symbiotic energy carriers, which can be electrochemically converted into each other by these electrolysers and fuel cells.
After the past steam era, the present combustion era, a sustainable energy era will emerge with new technologies.
Here comes the electro-hydrogen era!
Figures from: Ad van Wijk, Els van der Roest, Jos Boere, ‘Solar Power to the People’ Allied Waters, November 2017, ISBN 978-1-61499-832-7 (online)
Further reading about hydrogen, see website Ad van Wijk www.profadvanwijk.com
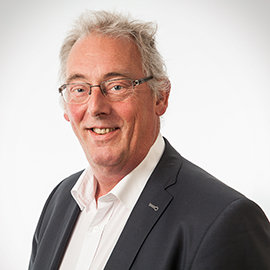
Prof. dr. Ad van Wijk
Ad van Wijk is part-time Professor Future Energy Systems at TU Delft, the Netherlands. He is guest professor at KWR Water Research Institute to develop and implement the research program Energy and Water. He is special advisor to Hydrogen Europe, representing European industry, national associations and research centers to develop European hydrogen policies with the EU commission. He is hydrogen ambassador at the ‘New Energy Coalition’ to realize the green hydrogen economy in the Northern Netherlands. And he holds several advisory and supervisory board positions.
Van Wijk has studied physics and did his Ph-D on Wind Energy and Electricity Production at Utrecht University in the Netherlands. He worked as an Researcher and Associate Professor, between 1983 and 1997 in the Department of Science, Technology and Society at Utrecht University
In 1984 Van Wijk founded the company Ecofys, which eventually grew into Econcern.
Econcern developed many new sustainable energy products, services and projects. Examples include the 120 MW offshore wind farm Princess Amalia in the North Sea, several multi-MW solar farms in Spain, a solar cell manufacturing plant Solland and a bio-methanol plant in the Netherlands.
Since 2011 Van Wijk is appointed as professor Future Energy Systems at TU Delft.
His research focus on the energy systems of the future. Especially he will do research on hydrogen energy systems and fuel cell cars and has realized ‘the Green Village’ at the TU Delft campus.
Van Wijk achieved many important prizes for excellent entrepreneurship. Amongst others he was Dutch entrepreneur of the year in 2007 and Dutch top-executive in 2008.
Van Wijk was honored by KWR, by appointing him Honorary Fellow in 2018.
Prof. dr. Ad van Wijk has published many books, scientific articles and reports. Amongst others:
‘How to boil an egg’, 2011 ISBN: 978-1-60750-989-9
‘Welcome to the Green Village’, 2013 ISBN 978-1-61499-283-7
‘Our Car as Power Plant’ 2014 ISBN 978-1-61499-376-6
‘3D printing with biomaterials’, 2015 ISBN 978-1-61499-485-5
‘The Green Hydrogen Economy in the Northern Netherlands’, 2017 ISBN 978-90-826989-0-9
‘Solar Power to the People’, 2017 ISBN 978-1-61499-832-7 (online)
‘Hydrogen, the key to the energy transition’, 2018 article in book
‘Hydrogen, the bridge between Africa and Europe’, 2019 article in book
‘A North Africa – Europe Hydrogen Manifesto’, 2019 DII desert energy report
‘Green Hydrogen for a European Green Deal; A 2×40 GW Initiative’, 2020 ISBN 978-90827637-1-3
All these publications can be downloaded from his website www.profadvanwijk.com
Follow Ad van Wijk at twitter @advanwijk or via his website.